-
-
News
News Highlights
- Books
Featured Books
- design007 Magazine
Latest Issues
Current IssueCreating the Ideal Data Package
Why is it so difficult to create the ideal data package? Many of these simple errors can be alleviated by paying attention to detail—and knowing what issues to look out for. So, this month, our experts weigh in on the best practices for creating the ideal design data package for your design.
Designing Through the Noise
Our experts discuss the constantly evolving world of RF design, including the many tradeoffs, material considerations, and design tips and techniques that designers and design engineers need to know to succeed in this high-frequency realm.
Learning to Speak ‘Fab’
Our expert contributors clear up many of the miscommunication problems between PCB designers and their fab and assembly stakeholders. As you will see, a little extra planning early in the design cycle can go a long way toward maintaining open lines of communication with the fab and assembly folks.
- Articles
- Columns
Search Console
- Links
- Media kit
||| MENU - design007 Magazine
Estimated reading time: 8 minutes
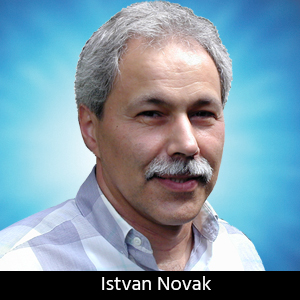
Quiet Power: What's the Best Method for Probing a PDN?
Recently, one of my friends asked about the preferred way of probing a power distribution network (PDN): “Which probe should I use to measure power plane noise? Assuming I am planning to measure PDN noise in time domain, I have two choices of probes: 1) a 50-ohm passive coax cable, or 2) an active high-impedance probe which is normally used for high speed signal measurement.”
Although, as usual, the correct answer starts with “It depends,” in this case the generic answer is more clear-cut: For many PDN measurements, a simple passive coaxial cable is better.
To determine which probe is better suited for time-domain PDN measurements, passive or active, low-impedance or high impedance, we have to consider three things: a) the signals we want to measure, b) the typical characteristics of oscilloscopes and c) the characteristics of passive and active probes.
First, let us look at the signals. As opposed to signal integrity measurements, where our signals tend to be much wider band and have a relatively large swing, typical PDN noise on a PCB is more band-limited and should be much smaller in magnitude. High-speed signal voltage swing is on the order of 1 Vpp at the transmitter, and it may be around 100 mVpp at the receiver.
In contrast, PDN noise on sensitive low-voltage power rails is measured in millivolts, at most a few times 10 millivolts peak to peak. Though newer signaling standards allow for very low voltage swing at the receiver, the contrast is clear: The magnitude range of the typical rail noise is lower than the typical range of signal swings. Luckily enough, the difference in bandwidth requirement helps us to make up for the differences in levels. Even after traveling through a lossy channel, the bandwidth of our SerDes signals has to extend up to at least the Nyquist frequency of signaling: half of the bit rate value.
For instance, the Nyquist frequency of a 5 Gbps PCIe Gen2 signal is 2.5 GHz. The majority of our PDN noise, on the other hand, goes through a chain of low-pass filters as it propagates between the board, package and silicon, resulting in a relatively band-limited signature on the board. The series connection impedance, together with the PDN impedances, creates a low-pass filtering on the order of 1 MHz for many of the memory sockets and high-power core rails, and tens, maybe hundreds of MHz cutoff frequency for lower-current power rails.
Unless we want to measure PDN crosstalk (noise coupled from one PDN rail to an adjacent PDN rail), which can be tricky in the time domain, or we want to measure high-frequency deterministic components, such as clock leakage to PDN (which can be better measured with a spectrum analyzer and not with an oscilloscope), we are better off using a lower-bandwidth oscilloscope for board PDN noise measurements.
Next, let us look at today’s oscilloscopes. We usually want to measure PDN noise in “real time,” without averaging. A big part of the PDN noise could be uncorrelated and random, and averaging would reduce both its peak and RMS value, which is exactly what we don’t want. We need real-time oscilloscopes, and we should possibly set them to infinite persistence mode to get the peak noise over time.
In recent years, the available bandwidth of real-time oscilloscopes has gone up significantly. Today, 30 GHz and 60 GHz real-time oscilloscopes are available [1], [2], [3]. However, to achieve such high real-time bandwidth, we have to accept lower vertical resolution and higher trace noise. The front-end raw quantization is limited to 8 bits. Moreover, the true number of bits of quantization, called the Effective Number of Bits (ENOB), drops in most cases to around 5 as the signal bandwidth extends to or beyond 10 GHz.
This in itself, on the other hand, may not matter too much for PDN noise measurements, because the precise shape of the noise waveform is usually less important. For PDN measurements, more limiting is the fact that the highest analog input sensitivity on wide-band real-time oscilloscopes is usually 10mV/div or worse, whereas real-time oscilloscopes with 1 GHz analog bandwidth may easily have 1 or 2 mV/div sensitivity. This tells us that medium-speed real-time oscilloscopes are best suited for today’s board-level PDN measurements. There are many good real-time oscilloscopes on the market with medium bandwidth. USB-powered hand-held oscilloscopes were used for the illustrations in this column [4], [5].
Finally, the probes. So far we have seen that we need only a limited bandwidth, but we need good sensitivity. Also, typical PDN impedances are much lower than the 50 to 150 ohms impedance range of high-speed signaling environment. Therefore, high-impedance probes are not necessary. High-impedance probes, passive or active, usually attenuate the signal, reducing the available sensitivity of the oscilloscope. The front-end amplifier of the active probe, unless very well shielded, may pick up unwanted signal from strong sources nearby, such as DC-DC converters. With active probes, we would need to worry about additional parameters as well – common-mode rejection, among others. All the above suggests that a direct coaxial cable connection is not only enough, but it is usually the best solution.
Finally, for any kind of probe, making good connections is crucial. We must minimize the size of the loop between the probe tips and the device under test.
To illustrate some of these concepts, we will look at the CPU core rail of a personal computer, measured with different probes and connection techniques. Figure 1 shows the personal computer motherboard and the location where the measurements were taken.
Figure 1. Multiphase core DC-DC converter on a personal computer board. The measurements were taken at the vacant capacitor site in the vertical column of bulk capacitors. This capacitor site was originally left unpopulated in manufacturing.
Two probes with three different connection techniques were used to collect the data. Figure 2 shows a 10:1 / 1:1 high-impedance passive probe with ground lead and clip. Figure 3 shows the same probe with the ground lead and clip replaced with a coaxial receptacle.
Figure 2. High-impedance passive probe with ground lead and clip.
Figure 3. High-impedance passive probe with coaxial receptacle.
The third option is shown in Figure 4: a piece of flexible 50-ohm coaxial cable (RG174 type) soldered to the vacant capacitor site on the back side of the board.
Figure 4. A 50-ohm coaxial cable soldered to the vacant capacitor site on the backside of the board.
The oscilloscope reading with the direct coaxial connection is shown in Figure 5. The coaxial cable is terminated in 50 ohms at the oscilloscope input. The peak-to-peak value is approximately 4 mV.
Figure 5. Oscilloscope reading with direct coaxial connection, computer turned ON.
When we use the passive high-impedance probe and connect it on the top side to the vacant capacitor site with the ground lead and clip as shown in Figure 6, we get the reading in Figure 7.
Figure 6. Connection of the high-impedance passive probe to the test site on the top side of the board.
Figure 7. Oscilloscope reading with the high-impedance passive probe in 1:1 setting, connected to the test site with the ground lead and clip as shown in Figure 6.
In this case the oscilloscope input impedance is left at 1 Mohm; the maximum reading is approximately 65 mVpp.
When we use the same high-impedance passive probe with a coax receptacle, connected to the vacant capacitor site on the back side of the board as shown in Figure 8, we get the reading shown in Figure 9. The maximum reading is approximately 16 mVpp.
Figure 8. High-impedance passive probe at the test site in coaxial receptacle.
Figure 9. Reading with the high-impedance passive probe in coaxial receptacle shown in Figure 8.
Why do we have such an enormous difference in the readings when, in all three cases, we connect the probes to the same capacitor site? To get the answer, we will need to do a series of reference and comparison measurements. We should start with the obvious: To rule out any external source of unwanted interference, we take the readings in each case with the computer turned OFF. In our case all three readings were a fraction of a millivolt, so this cannot be the issue.
The next check should be with the device under test turned on, and the probe electrically disconnected, but not removed. We can take a measurement with the probe tips open and shorted. With open probe tips, we check for electrostatic pickup of the probe, which is worse with high-impedance probes. The shorted-tip measurement checks for magnetic pickup from nearby sources, and it can be significant in active probes or in probes with large connection loops picking up close magnetic field from high-current DC-DC converter circuit loops.
We can also check for common-mode pickup through the probe and oscilloscope by connecting the shorted probe tips to the ground side of the test connections. Eventually, the measured voltage level will be a combination of the actual signal to be measured and these different erroneous noise pickups. When we do these reference measurements with the coaxial cable probe, all readings are negligibly small. With high-impedance passive probes in a coax receptacle, we get several millivolt erroneous readings. Finally, with high-impedance passive probes with ground leads and clips, the noise pickup is tens of millivolts. This is illustrated in Figure 10, which shows the noise pickup of the ground-lead loop of the passive high-impedance probe.
Figure 10. Magnetic noise pickup of the probe’s ground lead loop.
Bottom line: For power distribution noise measurements, use simple coaxial cables properly terminated at the oscilloscope input.
References
[1] LeCroy LabMaster 10Zi 65GHz real-time oscilloscope,[2] Agilent DSAX96204Q 63GHz real-time oscilloscope[3] Tektronix DSA70000 33GHz real-time oscilloscope[4] www.picotech.com/oscilloscope.html[5] www.tiepie.com/en
Dr. Istvan Novak is a distinguished engineer at Oracle, working on signal and power integrity designs of mid-range servers and new technology developments. Novak received his M.S. degree from the Technical University of Budapest, Hungary and his Ph.D. degree from the Hungarian Academy of Sciences in 1976 and 1989, respectively. With 25 patents to his name, Novak is co-author of "Frequency-Domain Characterization of Power Distribution Networks." To contact Istvan, click here.
More Columns from Quiet Power
Quiet Power: An Evolution in PCB Design CostsQuiet Power: The Effect on SI and PI Board Performance
Quiet Power: 3D Effects in Power Distribution Networks
Quiet Power: Noise Mitigation in Power Planes
Quiet Power: Uncompensated DC Drop in Power Distribution Networks
Quiet Power: Ask the Experts—PDN Filters
Quiet Power: Friends and Enemies in Power Distribution
Quiet Power: Be Aware of Default Values in Circuit Simulators